Environment & Energy
Related: About this forumHigh-Performance Engineered ZIF-67@PES Beads for Uranium Extraction from Aqueous Solutions
The paper to which I'll briefly refer in this post is this one: High-Performance Engineered ZIF-67@PES Beads for Uranium Extraction from Aqueous Solutions Krishan Kant Singh, Gourab Karmakar, Pallavi Singhal, Adish Tyagi, Amit Kanjilal, Kamlesh K. Bairwa, and Avesh K. Tyagi Industrial & Engineering Chemistry Research 2025 64 (14), 7548-7562.
In theory, albeit not in practice, it should not be necessary to mine uranium at all while still providing all of the world's primary energy using nuclear energy. This would involve converting all of the 238U now in reserve, known as "depleted uranium" to 239Pu (and higher isotopes) using a fast neutron spectrum. Alternatively, or perhaps in concert, recovering thorium from lanthanide (rare earth) mine tailings could extend these resources further.
The world inventory of depleted uranium is about 1.6 million tons. The energy content of a kg of fully fissioned 239Pu is about 80 trillion Joules. If we assume that world energy consumption can be stabilized at 650 EJ/year, perhaps with the judicious use of process intensification with heat networks, this suggests that mined and isolated uranium could last for about 250,000 years. (The caveat is a function of a parameter known as "doubling time, the time that depleted uranium must be in a neutron flux to be converted to plutonium.)
An alternative to mining uranium is to isolate it from aqueous solutions, most prominently seawater, an idea around which many thousands of papers have been written and published. The ocean contains about 4.5 billion tons of uranium, in dilute solution, part of a global uranium cycle involving the Earth's mantle, crustal uplift to mountains, weathering and transport by rivers to the sea. Uranium also naturally occurs in groundwater which is mined for water supplies.
An excellent monograph on this topic, which I have in my files is this one: S. Krishnaswami, J. Kirk Cochran Editors:U-Th Series Nuclides in Aquatic Systems (2008) Imprint: Elsevier Science. It has tables of concentrations of uranium in all of the world's major rivers.
Many of the aforementioned papers on extraction from seawater refer to a class of resins functionalized with amidoximes which have a high affinity for uranium.
The paper referenced above is different, the use of what are known as "MOFs," metal organic frameworks, the particular subclass being known as ZIF's ( Zeolitic imidazolate frameworks).
From the paper's introduction:
Solidliquid extraction can address these limitations and is a more practical approach to treating large volumes of waste containing low levels of uranium. Moreover, it is eco-friendly, economic, simple to use, and efficient in treating large volumes of waste streams. Various organic and inorganic materials have been synthesized and used as solid-phase sorbents to treat uranium-containing waste effluents. (9−13) Among them, polymer-based composites like beads, films, membranes, fibers, and nanocomposites are more promising and widely used to remove uranium from different waste streams due to their superior mechanical, chemical, and radiation stability. (1,2,12,14−18) However, despite these advancements, a key research gap remains in developing materials that combine a high uranium adsorption efficiency with structural robustness and ease of recovery for large-scale applications.
Metalorganic frameworks (MOFs) are a versatile class of novel porous materials, which have been extensively explored for energy and environmental remediation applications. (19−22) They show wide application in many fields, such as gas storage, (23) sensors, (24) catalysts, (25) and drug delivery. (26) Recently, MOFs have shown promising results for uranium recovery from water. This timely and crucial application of MOFs has led to an overwhelming number of scientific studies for uranium recovery through a number of MOFs such as HKUST-1, (27) MOF-76, (28) UiO-66, (29) MIL-101(Cr), (30) MIL-100(Fe), (31) UiO-66-NHPOPH2, (32) UiO-68, (33,34) and MOF Zn(HBTC)(L)(H2O)2. (35) This marks a significant step toward mitigating the treatment of radioactive waste and also recovery of uranium from seawater. Zeolite imidazolate frameworks (ZIFs), a promising member of the MOF family, have shown potential for the adsorption of heavy metals...
The authors refer to a particular ZIF, ZIF-67, which, addressing the problem that it is in a powder form. To address this issue, the authors suspend the ZIF-67 in a polysulfone polymer.
Some graphics from the paper:
A cartoon on the synthesis:
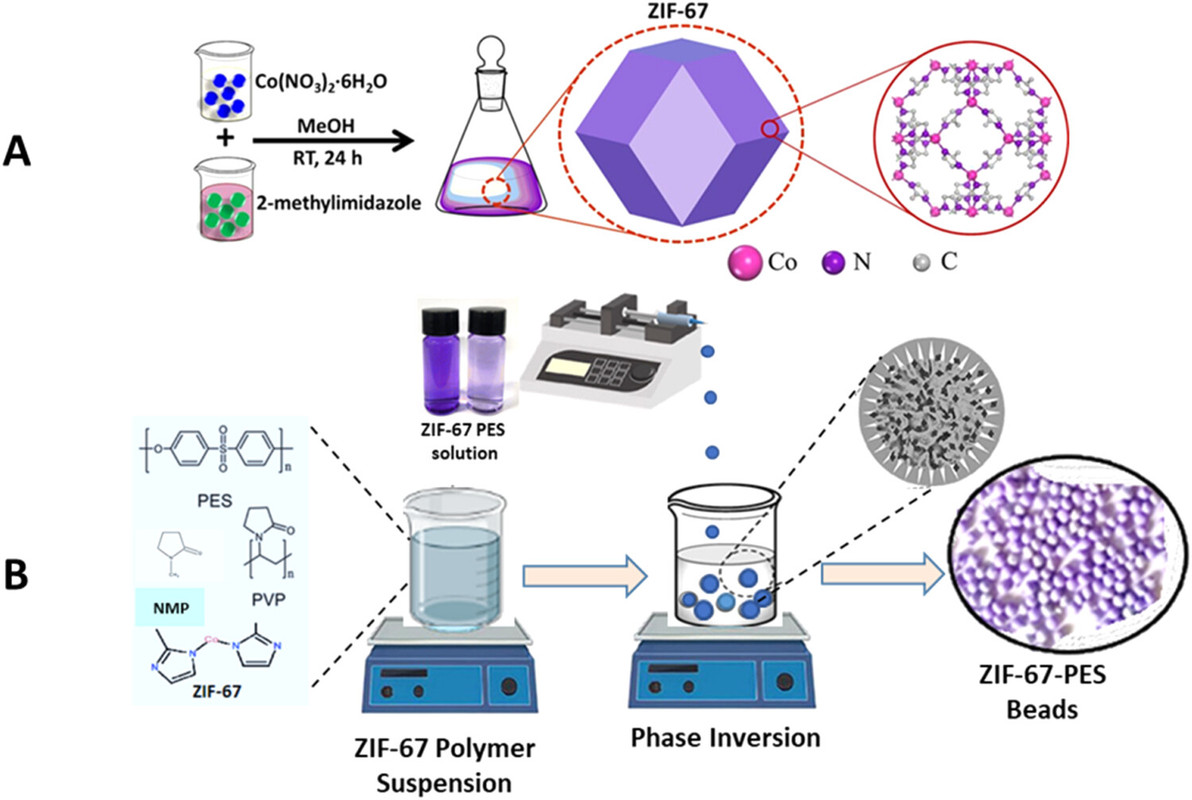
The caption:
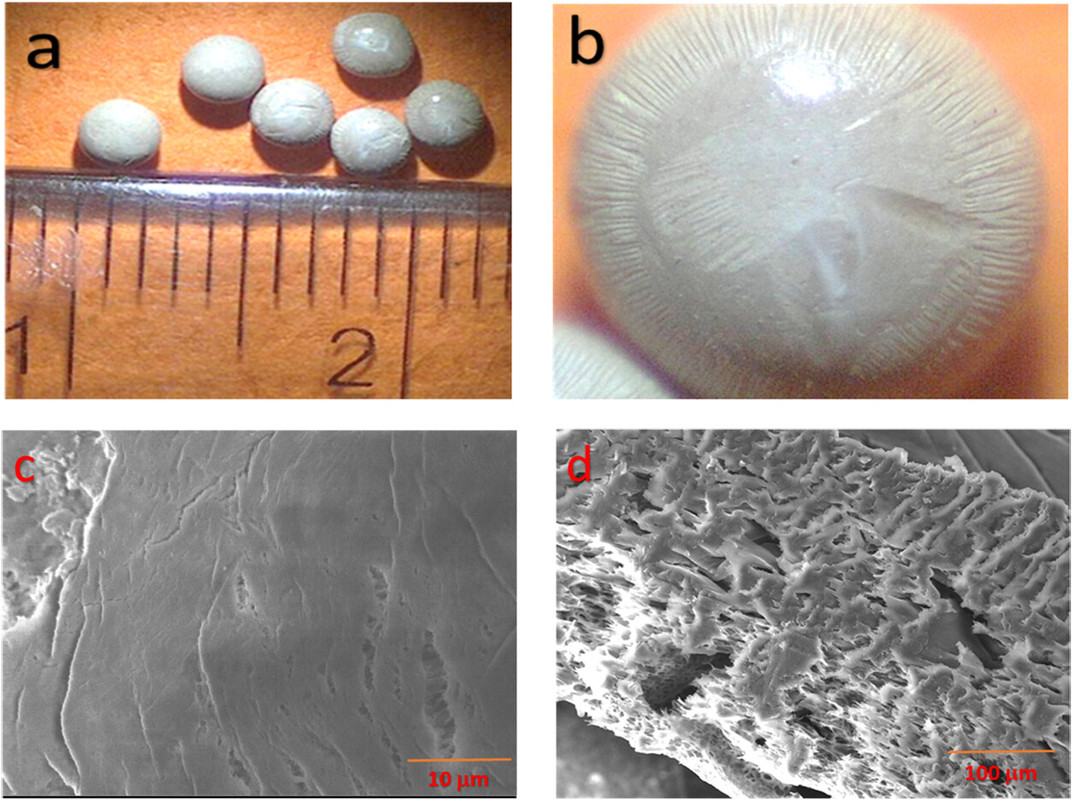
The caption:
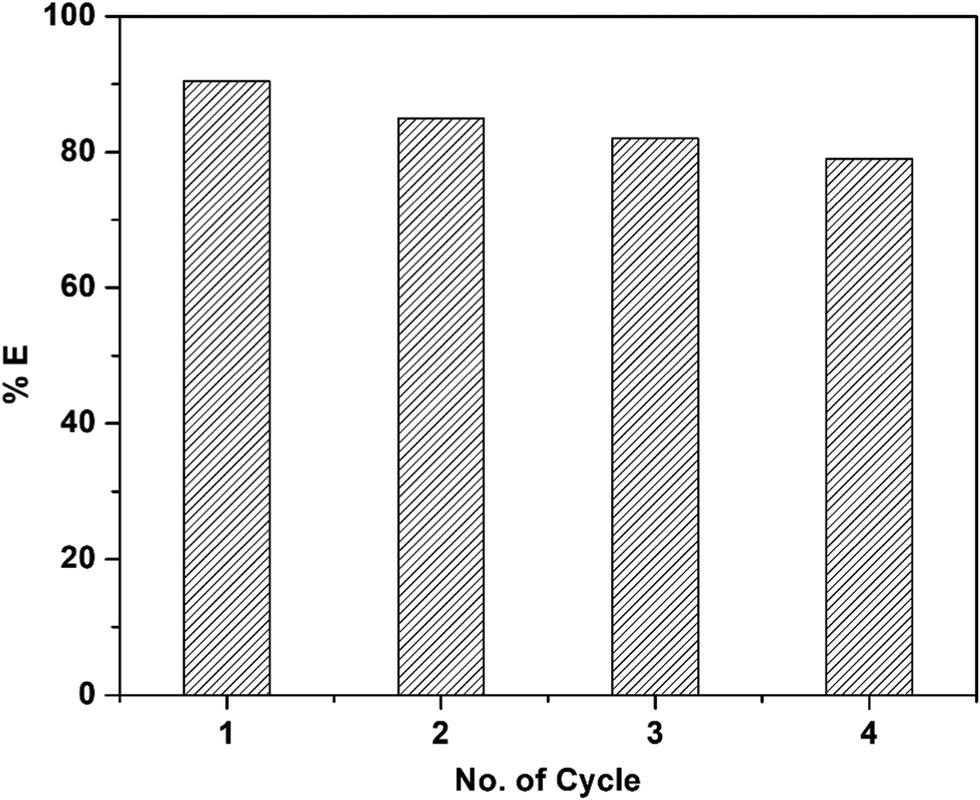
The caption:
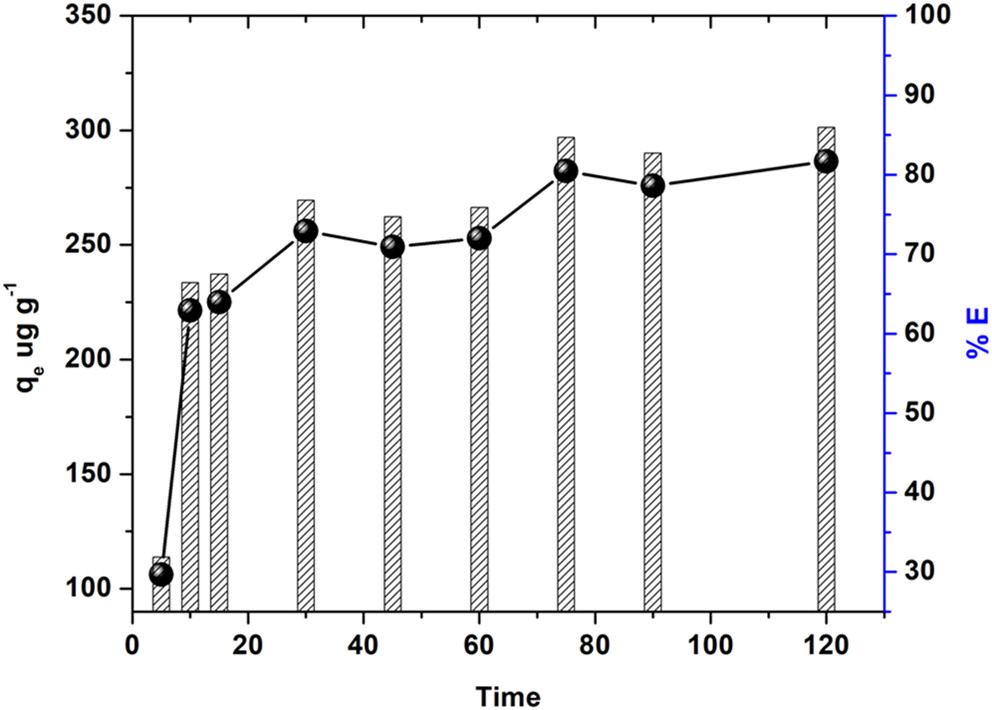
The caption:
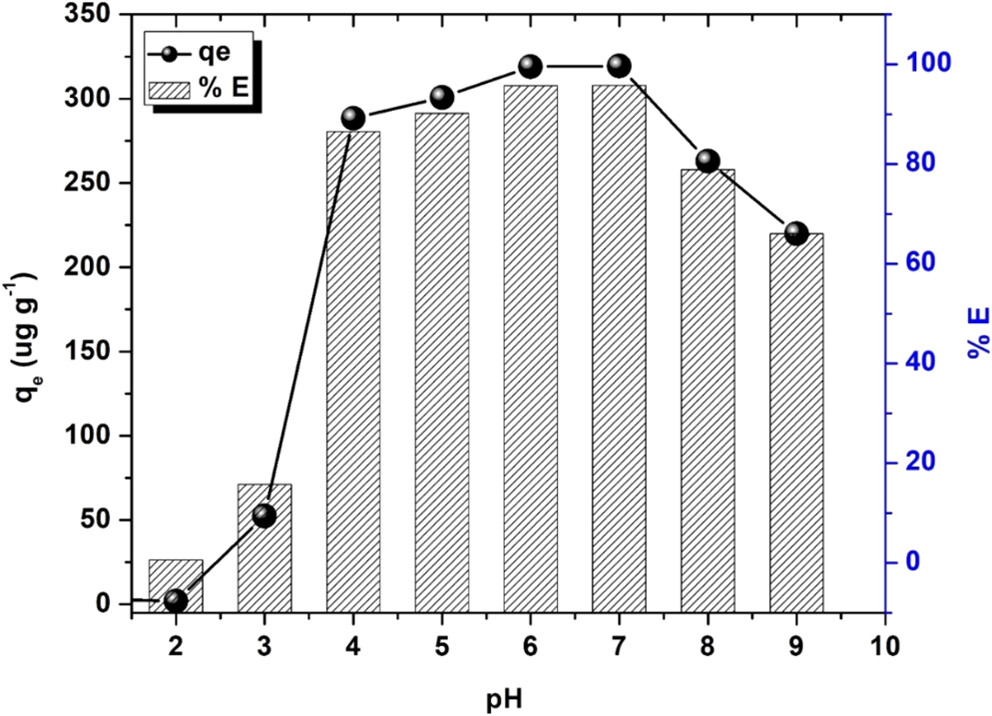
The caption:
The paper's conclusion:
Again, the recovery of uranium from seawater (which is currently more expensive than mined uranium) conceivably might not be necessary for hundreds of thousands of years, although such recovery might be a side product of removing natural uranium from drinking water and other related sources. The reprocessing of used nuclear fuel relies on solvent extraction which can lead to uranium contaminated water, but personally I would choose to supplant solvent extraction with fluoride volatility methods. Therefore this approach can be used to clean up process water, as well as runoff from abandoned uranium mines.
It is hardly the only approach, but to my knowledge is somewhat unique, although outside of my purview, the use of ZIF-67 for uranium capture has been known for some time. This is a nice spin on the idea, in my view.
Have a nice day tomorrow.